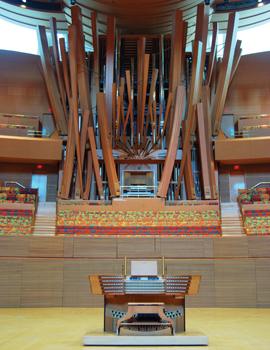
Solid State Organ Systems announces that the latest software for MultiSystem II and Capture for MultiSystem II has been installed in the Glatter-Götz Rosales organ at Walt Disney Concert Hall, Los Angeles, California. The project has been managed by Manuel Rosales of Rosales Organ Company. The installation controls both a mechanical-action main console and a remote, movable stage console.
The Solid State Organ Systems MultiSystem II now features wireless control for record/playback, wireless tuning, full MIDI compatibility, and Organist Palette with over 50 organist Libraries.
For information: 703/933-0024, [email protected], www.ssosystems.com/.