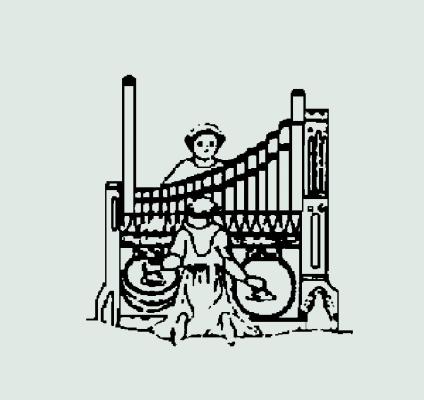
Thar she blows—some more
While writing my column last month I ran out of space and had plenty of air left, so today I continue my stream-of-consciousness about organ wind. (You might want to reread the April typhoon first.) I ended last month with a literary reference—let’s start this month with another, this time from American poet Oliver Wendell Holmes (1809–1894):
The Organ Blower
Devoutest of my Sunday friends,
The patient Organ-blower bends;
I see his figure sink and rise,
(Forgive me, Heaven, my wandering eyes!)
A moment lost, the next half seen,
His head above the scanty screen,
Still measuring out his deep salaams
Through quavering hymns and panting psalms.
No priest that prays in gilded stole,
To save a rich man’s mortgaged soul;
No sister, fresh from holy vows,
So humbly stoops, so meekly bows;
His large obeisance puts to shame
The proudest genuflecting dame,
Whose Easter bonnet low descends
With all the grace devotion lends.
O brother with the supple spine,
How much we owe those bows of thine!
Without thine arm to lend the breeze,
How vain the finger on the keys!
Though all unmatched the player’s skill,
Those thousand throats were dumb and still:
Another’s art may shape the tone,
The breath that fills it is thine own.
Six days the silent Memnon waits
Behind his temple’s folded gates;
But when the seventh day’s sunshine falls
Through rainbowed windows on the walls,
He breathes, he sings, he shouts, he fills
The quivering air with rapturous thrills;
The roof resounds, the pillars shake,
And all the slumbering echoes wake!
The Preacher from the Bible-text
With weary words my soul has vexed
(Some stranger, fumbling far astray
To find the lesson for the day);
He tells us truths too plainly true,
And reads the service all askew,—
Why, why the—mischief—can’t he look
Beforehand in the service-book?
But thou, with decent mien and face,
Art always ready in thy place;
Thy strenuous blast, whate’er the tune,
As steady as the strong monsoon;
Thy only dread a leathery creak,
Or small residual extra squeak,
To send along the shadowy aisles
A sunlit wave of dimpled smiles.
Not all the preaching, O my friend,
Comes from the church’s pulpit end!
Not all that bend the knee and bow
Yield service half so true as thou!
One simple task performed aright,
With slender skill, but all thy might,
Where honest labor does its best,
And leaves the player all the rest.
This many-diapasoned maze,
Through which the breath of being strays,
Whose music makes our earth divine,
Has work for mortal hands like mine.
My duty lies before me. Lo,
The lever there! Take hold and blow!
And He whose hand is on the keys
Will play the tune as He shall please.
Such an eloquent daydream! Holmes was a doctor of medicine and held a chair of anatomy and physiology at Harvard for most of his working life.1 He has us in a church with “shadowy aisles,” but I picture him sitting in a white New England church with lots of clear glass, a little woozy from the bright sunlight. There’s a black-walnut organ case up front behind the pulpit, and the pump-handle sticks out the right-hand side of the case. Perhaps our dreamer missed a brilliant sermon that morning, but he seemed not to hold the preacher in high esteem: He tells us truths too plainly true, and reads the service all askew . . . Instead we get a rare glimpse at 19th-century worship in which we see the organ-pumper as a participant in the service, “scanty screen” notwithstanding. I’ve never designed or built a new organ with a manual pumping system. I would have imagined that I would try to place the pump handle out of sight so the motion wouldn’t detract from the worship, but perhaps that would deprive the congregation from deeper insight into the Word of God. The pump handles of many of the antique organs I know stick out of the side of the instrument where the motion of the pumping would have been quite a spectacle. I wonder how many worshipers made the connection between the volume of the music and the speed of the pumping?
The largest single part of most 19th-century American pipe organs is the reservoir. Recently I was inspecting a large Hook organ in New York City as the Organ Clearing House prepares to dismantle it, and I measured the reservoir at 12' x 6', double rise, with two feeder bellows underneath, each of which is half the size of the main reservoir. (In this organ, the pump handle was inside the case.) I was looking at it from a logistical point of view—the OCH crew will soon have to lift it out of the organ loft—but as I like to imagine the organ as a living, breathing entity, this enormous and heavy mechanism is one of the organ’s vital organs. If the reservoir is 12' x 6' and opens 18" when full of air, it has a capacity of about 108 cubic feet. The feeders open about a foot and are wedge-shaped—as they each take up half the area of the reservoir, each has a capacity of about 18 cubic feet. The pump-handle pivots between the two feeders—when the handle goes up, one feeder opens and the other closes so one cycle of the pump-handle (up and down) feeds 36 cubic feet into the reservoir—assuming no leaks, it takes three strokes to fill the reservoir. Right? Read on.
Fill the reservoir and then stop pumping. Play a hymn on one stop. You’ll get through a whole verse, maybe more, before the bellows is empty. Pump it up again and play the same hymn on full organ. This time you’ll run out of air before you finish the first line. You might have to pump twice a measure to keep air moving at full organ. How’s that for scientific?
With few exceptions, the case (especially the footprint) of a 19th-century organ is much larger than that of a modern organ with the same number of ranks. Why? I’ll give you one reason. Walk around the modern organ case and you’ll find the reservoir mounted on a frame behind the organ. The footprint of the 19th-century organ is established by the size of the reservoir located inside the case.
Most 19th-century instruments have a service access door at ground level which means that the first thing a visitor sees inside the organ is the reservoir. Actually, what they see is an ocean of bricks stretching into the darkness and they always ask why an organ needs bricks. The weight of the bricks creates the pressure. Forcing air into an elastic reservoir (an organ bellows with hinged ribs) will not create pressure until we add weight to the top of the reservoir. The amount of weight determines the level of pressure—add weight and the pressure increases.
One colleague of mine made it a practice to use indigenous materials to weigh the bellows in the instruments he built. One organ was near a granite quarry, another, marble. One was near old shoe-making factories so they used the cast-iron heel molds.
I said that three strokes of a 36-cubic-foot pumping cycle would fill a reservoir that holds 108 cubic feet. Wrong! To put air under pressure you compress it. So it takes many more than three strokes of atmospheric pressure to fill that reservoir. (That math is beyond me!)
Bricks used as reservoir weights are often wrapped in paper. Why go to all that trouble? Bricks are porous and can absorb moisture from the air, which increases their weight, and the paper inhibits absorption. The organ is tuned and voiced at a specific pressure. If the pressure goes up too much, the sound of the organ will be compromised. Imagine the reaction of the organ tuner when he arrives at the church and finds a stack of folding chairs stored on top of the reservoir!
The floating top frame of the reservoir with all its bricks is very heavy—you can’t budge it. But the organ’s wind lifts it effortlessly. And when it’s full, a touch at one end makes the whole thing rock gently—a wonderful illustration of both the power and the delicacy of this musical air. Our friend the organ-pumper can move mountains with his pump handle. There are few natural forces more powerful than air. An airliner overshoots the end of the runway, the landing gear collapses, and emergency workers lift the plane with huge inflatable bags placed under the wings. Air moving fast across the countryside (wind) blows the roof off a barn. You stand on the platform of a railway station and an express train roars through—the blast of air pushed aside by the locomotive almost knocks you over. Or sit in a sailboat at noon on a calm sunny day. As you glide gently along the glassy water you notice a line of rough water a thousand yards away moving toward you. The heat of the sun has warmed the land. The air above the land is rising, and the air above the cooler water is rushing ashore to fill the void. The wind is caused by air being drawn, not blown. (A barometer measures atmospheric pressure—a falling barometer is an indication of coming wind—a fast falling barometer indicates an impending storm.) The wind is above the surface so your sail is filled before the rough water gets to you. The boat heels and the water bubbles out from under your stern as you race across the water. Does the blowing wind push the boat along? If that’s all it could do, then the boat could only move in the same direction as the wind. The curve of the sail is the exact equivalent of the curve of the top of an airplane wing, turned ninety degrees from horizontal to vertical. The plane is pushed forward by its engines. Since the curved top of the wing is a longer distance to cover than the flat bottom, the air on top of the wing moves faster. The faster moving air creates a lower pressure above the wing than below, and the plane lifts toward the lower pressure. The curve of the boat’s sail makes the wind move faster across the front of the sail than the back, and the boat is drawn forward. The racing sailor’s jargon includes the word lift which refers to a gust of wind. I got lifted to the first racing mark.
As I visit organbuilders’ workshops, I’ve noticed with both pleasure and amusement how common it is to find half-finished sailboat parts (rudders, tillers, etc.) stored under the workbenches; the employees’ weekend projects mix woodworking with wind. There is a strong correlation between sailboats and pipe organs. In my interpretation, it’s no accident that the logo of C. B. Fisk, Inc. (organbuilders in Gloucester, Massachusetts) is the masts, yards, and rigging of a square-rigged sailing ship.
When you play four verses of a hymn on a large organ you send 10,000 cubic feet of pressurized air (2500 ft3/minute x 4 minutes) out of the blower, through the reservoirs, through the pipes, and into the sanctuary converted into sound energy. I don’t believe speakers can duplicate that.
Today, we slide onto the organ bench and flip a switch. An electric motor comes on turning a fan that blows air through ducts into the reservoirs. When the fast-moving air is contained by the reservoir with weights (or springs) pushing down on its top, pressure is created, regulated, and stored until you are ready to use it by playing. In a large organ, the blower is a huge machine hidden in a remote location. It might be the size of a small car and have a 10, 20, or even 30 horsepower motor. Many people never throw the switch that turns on a machine that large. Among other industrial innovations, the development of the jet engine has resulted from research about the nature of moving air so modern blowers can be much smaller and quieter than the older monsters that lurk in church basements. It’s common for a newer blower to be installed right inside the organ. This means less work and expense building windlines, and it means that the organ pipes are sitting in the same atmosphere that’s being used to blow them. When an organ blower sits in a cold basement room, the cool air blowing through the warm pipes upsets the tuning. And remember our 10,000-cubic-feet-per-hymn; think of the waste of heating fuel when you blow that much basement air into a heated sanctuary.
The organ blower is a great convenience. Imagine if scheduling organ-pumpers were added to the more familiar chores of the modern church organist. But don’t take that blower switch for granted. Think of all that grand air rushing through your instrument, converting to sound energy as it goes through the pipes, blending with the body of air-driven sound coming from the lungs of the congregation. It’s a winning combination.
One Saturday morning I received a frantic call from the organist of a church whose organ I maintain. A wedding was about to start and the organ wouldn’t work. She could hear that the blower turned on and the console lit up the way it always does, but no sound anywhere. I rushed to the church to find limousines lined up out front, and photographers running around. The church was full, and the bagpipe was vamping (egads!) to fill the time. Sure enough, the blower was running and the console was lit (so I knew that the power supply was on), but the bellows hadn’t risen—there was no air pressure in the organ. I ran to the basement where I found a card table resting against the organ blower’s air intake. That’s all it took. No air, no music. Can a card table stop bagpipes?