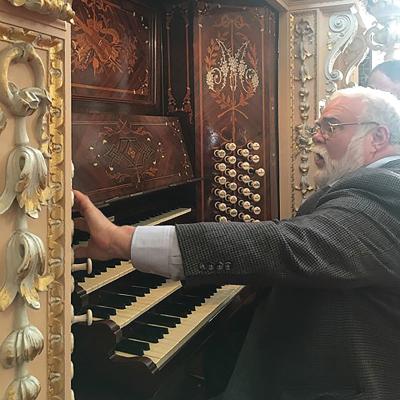
Shifty and puffy
It is mid-September in mid-coast Maine, and the days are getting shorter. Sunset here is about sixteen minutes earlier than in New York City, as we are as far east as we are north of the Big Apple. There are four windows facing east in our bedroom that allow us to track the motion of the sun, which is rising further south than it did a month ago. When we are on the water, we notice that the afternoon sun is lower in the sky as the sunlit water sparkles differently than in the height of summer. And the wind changes dramatically with the change of season. In mid-summer, we cherish the warm sea breeze, predominant from the south or southwest, caused by the air rising as it crosses the sun-warmed shore. All that cooler air above the ocean rushes in to fill the void, and we can sail for miles without trimming the sails in the steady and sure wind.
We had our last sail of the season last weekend in lumpy, bumpy wind from the northwest, which is never as steady as the southwesterlies. It is shifty and puffy, and it can be a struggle to keep the boat going in a straight line. Just as you get going, you get “headed” by a burst of wind from straight ahead, or you get clobbered abeam by a twenty-five mile-per-hour gust. Oof.
You have read this kind of thing from me before, thinking about sailboats when I should be writing about pipe organs, but because both are important parts of my life, and both involve the management of wind, I cannot escape it. And I am thinking about it a little more than usual because at the moment I am releathering three regulators for the organ I am working on. My method for assembling and gluing the ribs and frames of a wind regulator involves seven steps:
• Glue outside belts on the pairs of ribs.
• Glue inside canvas hinges on the pairs of ribs.
• Glue canvas hinges around regulator frames and bodies.
• Glue ribs to top frames.
• Glue ribs/top frames to body.
• Open regulator and glue gusset bodies.
• Close regulator and glue gusset tails.
It is still officially late summer as I write this, and my personal workshop is a three-car garage. Since we are on the shore, I love to have the overhead doors open to the breezes, though it is humid here. I am using the traditional flake hide glue (the stuff that is made when the old horse gets sent to the glue factory) that you cook in an electric pot with water, apply hot, and wipe clean with a hot-water rag that I keep just hot enough that I can put my hands in to wring the rag dry in the sort of double-boiler from which you scoop oatmeal at a cafeteria line. For the glue to set, the moisture must evaporate, and since the air is humid, I have to wait overnight between each step. Running fans all night keeps the humidity down and speeds the drying. In winter, when the air inside is dry, I can typically do two gluing steps in a day.
One of the regulators I am working on is thirty inches square. For that one I am using around twenty-five feet of one-inch-wide heavy canvas tape for the hinges and a comparable length of laminated rubber cloth for the outside belts. The gussets (flexible leather corner pieces) are cut from supple heavy goat skins that have a buttery texture and are impossible to tear. The key to finishing a wind regulator is finding a combination of materials that are all very flexible and strong, that are easy to cut, and that receive glue well enough to ensure a really permanent joint. If the structural integrity of a regulator is iffy, the wind will be shifty and puffy, and it will be a struggle to keep the music going in a straight line. Just as you get going, you get “headed” by a burst of wind that jiggles the music, or you get clobbered by a jolt from out of nowhere.
What’s in a name?
I am referring to these essential organ components as “regulators.” We also commonly call them “bellows” or “reservoirs.” All three terms are correct, but I think regulator is the most accurate description of the function of the thing. Taken literally, a bellows produces air. Air is drawn in when it is opened and pushed out when it is closed, like the simple bellows you have by the fireplace. The hole that lets the air in is closed by an internal flap when air is blown out.
A reservoir stores air. In an organ built before the invention of electric blowers, it was common for an organ to have a pair of “feeder bellows” operated by a rocking handle that blew air alternately into a large reservoir. The feeders had the same internal flaps as the fireplace bellows. The top of the reservoir was covered with weight (bricks, metal ingots, etc.) to create the air pressure, and the air flowed into the organ as the organ pipes consumed it. The bellows were only operated, and the reservoir was only filled when the organist was playing. Just try to get that kid to keep pumping through the sermon. . . .
With the introduction of the electric blower, it became usual to turn the blower on at the beginning of a concert or service and leave it running. That made it necessary to add a regulating valve between the blower and the reservoir. When the reservoir filled and its top rose, the valve closed, stopping the flow of air from the blower, so the system could idle with the blower turning and the reservoir full. When the organist played and therefore used air, the top of the reservoir would fall, the valve would open, and the air could flow again. Like before, there was weight or spring pressure applied to create the proper wind pressure. The addition of that valve added the function of pressure regulation to the bellows. In an organ with an electric blower, the bellows are storing and regulating the pressurized air. Calling it a regulator seems to cover everything.
The longer you go, the heavier you get.
Twice in my life, I have heard EMTs comment about my weight when lifting the stretcher, once after a traffic accident in the 1970s, and again after a fall in an organ seven years ago. But that is not what I am talking about here. We usually think of an inch as a unit to measure length or distance, so how can it refer to pressure, as in, “the Swell division is on six-inches of pressure?”
In industrial uses of pressurized air, more familiarly, in the tires or of your car, the unit of measure is pounds per square inch (PSI). I inflate the tires of my car to 35 PSI, and I use 80 or 100 PSI to operate pneumatic tools. But while my workshop air compressor gauges those high pressures, the actual flow is pretty small, something like two cubic feet per minute.
Organ wind pressure is much lower, and we measure it as “inches on a water column.” Picture a clear glass tube in the shape of a “U” that is twenty-inches high. Fill it halfway with water, and apply pressure to one side of the U. The water goes down on that side of the tube, and up on the other. Use a ruler to measure the difference, and voilà, inches on a water column, or centimeters, or feet. You can easily make one of these using plastic tubing. The little puff it takes to raise three inches of pressure is just the same little puff it takes to blow an organ pipe you are holding in your hand. Instead of the actual tube full of water, we use a manometer that measures the pressure on a gauge without spurting water onto the reeds.
Did you ever wonder how the conversion works? One PSI equals almost 28 inches on a water column. Five inches on a water column equals about .18 PSI. And how does that relate to the organs you know? In a typical organ, it is usual to find wind pressures of three or four inches. In general, smaller organs with tracker action might have pressures as low as forty millimeters, or less than two inches. In a three-manual Skinner organ, the Great might be on four inches, the Swell on six, and the Choir on five. In a big cathedral sized organ, solo reeds like French Horn and English Horn might be on fifteen inches, while the biggest Tubas are on twenty-five. The world-famous State Trumpet at the Cathedral of Saint John the Divine in New York City is on fifty inches (incredible), and in the Boardwalk Hall organ in Atlantic City, New Jersey, the Grand Ophicleide, Tuba Imperial, Tuba Maxima, Trumpet Mirabilis are on one hundred inches of pressure, or 3.61 PSI! Stand back. Thar she blows!
Once you have determined pressure, you also have to consider volume. A twenty-rank organ at three inches of pressure might need 1,000 cubic feet per minute at that pressure to sustain a big chord at full organ. Some of the largest organ blowers I have seen are rated at 10,000 CFM at ten inches of pressure. And when you lift the biggest pipe of a 32′ Open Wood Diapason and play the note as an empty hole, you will blow your top knot off. It takes a hurricane coming through a four-inch toehole to blow one of those monster organ pipes.
All the air you could wish for
Before the introduction of the electric blower, most organs had at least two bellows. One would be in free fall, supplying pressure to the organ while the other was raised by the organ pumper. The system I described earlier with two feeders and a reservoir was a great innovation, because once the reservoir was full, the pumper could slack off a little if the organist was not demanding too much wind. The six-by-nine-foot double-rise reservoir in the heart of a fifteen-stop organ by E. & G. G. Hook or Henry Erben has huge capacity, and can blow a couple 8′ flutes for quite a while without pumping. Organs by Hook are great examples of efficiency, with pipes voiced in such a way as to produce lots of tone with very little air, and even large three-manual organs are pumped by just one person using the two-feeders-and-a-reservoir system.
The electric blower changed everything. Organbuilders and voicers could now work with a continuous flow of wind at higher pressures than were available before. New styles of voicing were invented, and along with the introduction of electric keyboard actions, organs could be spread around a building, creating stereophonic and antiphonal effects. When organs were first placed in chambers, and their sounds seemed remote, the builders raised the pressure and increased the flow of air through the pipes, driving the sound out into the room.
While modest organs with electric blowers usually have only one wind regulator, larger instruments can have dozens. In a big electro-pneumatic organ, it is common to have a separate regulator for each main windchest. That is how Ernest Skinner could have the various divisions of an organ on different wind pressures, as each individual regulator can be set up to deliver a specific pressure.
But what about wiggly?
When I mention factors that can add to the stability of an organ’s wind system, I raise the question about “wiggly wind,” or “shaky wind,” both somewhat derogatory terms that refer to the lively flexible wind supplies in smaller and mid-sized mechanical action organs with lower wind pressure. When wind pressure is low and an entire organ receives its air from a single regulator, the motion of the wind can be affected by the motion of the music. It is especially noticeable when larger bass pipes are played while smaller treble pipes are sustained. At its best, it is a delightful affect, akin to the natural flow of air through the human voice. At its worst, it is a distraction when the organ’s tone wobbles and bounces.
This phenomenon is part of the fierce twentieth-century debate concerning “stick” organs versus so-called “industrial-strength” electro-pneumatic organs. I have been servicing organs for more than forty years, and I have often thought that much of the criticism of the emerging tracker-action culture was because craftsmen were reinventing the wheel, learning the art of organbuilding from scratch. They may have measured the dimensions of an organ bellows accurately but failed to compensate for the fact that the ancient model did not have an electric blower. And let’s face it: a lot of flimsy plywood tracker organs were built in the 1960s and 1970s, enough to give that movement a bad name from the start.
The evolution of modern tracker organs toward the powerful, thrilling, reliable, sonorous instruments being built today has much to do with how much the craft has learned about the management of wind over the years. A little tracker organ built in 1962 might have key channels and pallets that did not have the capacity to blow their pipes. It might have flexible wind conductors to offset bass pipes that were too small and that jiggled when the notes were played, causing the tone to bounce. It might have bass pipes with feet that were too short, so air did not have a chance to spread into a dependable sheet before passing between the languid and the lower lip. All of these factors affect the speech of the pipes, giving the impression that the organ is gasping for air. And worse still, you might hear the pitch drop each time you added another stop. I have worked on organs where adding an 8′ Principal made the 4′ Octave sag. How do you tune a thing like that? I marvel now at how air pressure moves through the best new tracker organs, especially at the wonderful response of large bass pipes. Organs by builders like Silbermann do not lack in bass response. Once the revival movement was underway in the middle of the twentieth century, it took a few decades to really start getting it right.
§
The organ I am working on today is a simple little thing with two unit action windchests. Each has its own regulator, and there is a third “static” regulator that mounts next to the blower. The blower produces seven inches of pressure; the static regulator brings it down to five inches and distributes the wind to the other two regulators, which each measure out four inches. The biggest pipes in the organ are the 16′ Bourdon, and though there are only ten ranks, it is a unit organ, and a lot of pipes can be playing at once. It is destined to be a practice instrument for a university organ program, so I know that talented and ambitious young organists will be giving it a workout as they learn the blockbuster literature we all love so much. I hope that those students never have to worry about having enough air. And perhaps Maine’s salty breezes will travel with the organ, adding a little flavor to the mix.