Since 1968, Francesco Ruffatti has been a partner, along with his brother Piero, of Fratelli Ruffatti—Ruffatti Brothers Family of Artisans—of Padova, Italy. The firm is involved in the restoration of historic organs and the construction of new pipe organs, and has worked for decades in Italy and many other countries, including the United States, Canada, South Korea, Japan, Australia, Mexico, and Sweden. Francesco Ruffatti holds the position of tonal designer with the company. He supervises the design of construction parameters of the pipe stops and their voicing. He is involved directly in the study, cataloguing, and restoration of voicing, and researching the temperament of the pipework of ancient organs undergoing restoration. He has co-authored several publications and has written articles in this area of expertise for both Italian and American journals, including “Gaetano Callido, Organbuilder in Venice,” The Diapason, December 1998, and “The Historical Italian Organ—Tradition and Development,” The Diapason, June 2001. He has also participated as a speaker at numerous conferences. A two-term past president of the Association of Italian Organbuilders, Francesco Ruffatti is currently teaching restoration practices and the theory and practice of flue and reed voicing at the school for organbuilders of the Lombardy region in Crema, Italy. Fratelli Ruffatti is a member of both the Association of Italian Organbuilders and the International Society of Organbuilders. Judit Angster comes from the famous Hungarian organbuilder family Angster. She holds a Diploma and PhD in physics. Since 1986, she has been engaged primarily in pipe organ research. Since 1992, she has been working for the Fraunhofer Institute (IBP) in Stuttgart, Germany, as head of the “Research Group of Musical Acoustics,” where, among other things, important European research projects were carried out in close cooperation with organ building companies. From 1994 until 2003, she taught classes in acoustics for master craftsman courses (the highest level of education and training) for organ building at the Federal College of Organ Building in Ludwigsburg, and intensive advanced training courses for pipe organ and church acoustics at the Fraunhofer Institute (regular workshops for further education of organ experts). She also lectures in acoustics at the University of Stuttgart and at the University of Music and Fine Arts in Stuttgart. Dr. Angster is President of the Technical Committee of Musical Acoustics of the German Society of Acoustics (DEGA) and a member of the Executive Board Council of the German Society of Acoustics (DEGA). She is the author of 115 publications in scientific/technical journals, conference proceedings, etc., ten patents, one book, 113 invited papers for conferences, congresses and at different institutes and societies.
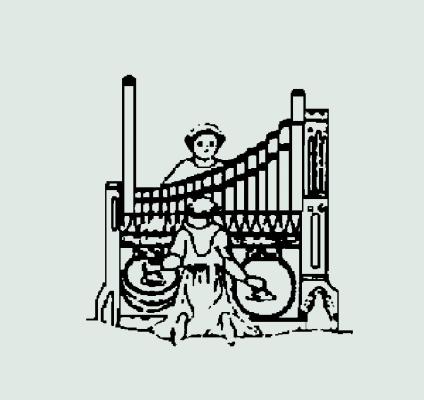
The organbuilder’s viewpoint
Investing in research is foreign to most organbuilders. The pipe organ is a traditional instrument, for which it is natural to think that everything has already been invented. Research is therefore perceived by most as something that has no value, since no advancements can be made. The possible exception to this involves the console systems and controls, where conservative attitudes in many cases have been overwhelmed by the very practical need of many organists to have tools on hand that can facilitate their performances. But what about sound, and the very principles that control the ancient art of voicing? In such areas, one will find that every single pipe voicer thinks that his way is the way it should be done, and procedures cannot be improved upon from his normal practices.
Voicing is largely a matter of taste, and subjective preferences are the only governing factors. Very often, an organbuilder is chosen because of the sound that his instruments produce, meaning essentially the stylistic approach to sound that he takes. Why would he then be interested in research in this field? Why change something that already works?
My entire career has been guided by two principles: anything can be improved upon, and an organbuilder never ceases to learn. The combination of these two beliefs has determined my personal desire to take part in scientific research programs. For almost a decade, Fratelli Ruffatti has participated in joint European projects aimed at finding ways to improve the art of organbuilding. Such projects have determined the need to conduct a great deal of fundamental research, which has been carried out over the years by a number of notable institutions, among which are the Fraunhofer Institut für Bauphysik (IBP) in Stuttgart, Germany, the University of Edinburgh, the University of Prague, the University of Budapest, and the Steinbeis Transfer Center of Applied Acoustics in Stuttgart. The Fraunhofer IBP in particular has been the constant guide and the main force behind fundamental and applied research.
The programs have been encouraged and co-sponsored by the European Commission in Brussels. A small group of organbuilders,1 coming from different European countries, participates in the research investment and actively cooperates with the scientists. Astonishing results have been obtained over the years, ranging from more efficient and silent wind systems, to efficient ways to evaluate room acoustics and to better adapt pipe organs to different acoustical environments. Recently, a revolutionary wind system has been invented, a monumental advancement over the traditional winding methods, which allows the organbuilder to simply avoid the use of reservoirs, schwimmers or related equipment, while at the same time obtaining unprecedented stability and efficiency in the wind supply of pipe organs.
The research currently under way deals with sound. The aim of this two-year process is to find better ways to reduce or eliminate problems that exist both in the field of “scaling,” or pipe dimensioning, and in “voicing,” meaning the process by which the pipes are given their proper sound character. At first sight, one may think that a project of this nature is aimed at “standardizing” organ sound by promoting uniform procedures for all. This is not at all the case. The idea is to provide scientific, undisputable knowledge, which can be used by each organbuilder to better reach his individual tonal ideals. Examples are the application of scientific principles to calculate an efficient shape for large wooden pipes that will make them prompt in their attack despite their size, while ensuring the production of the needed fundamental. Other interesting examples under research are finding practical ways to make the transition between stopped and open pipes, or the transition between wooden and metal pipes within one single rank, as tonally undetectable as possible.
In such a research program, the subject of voicing techniques could not be avoided. Once again, the objective was not that of teaching new ways to voicers with decades of experience, but to find out scientific evidence in a field that has never been properly analyzed with scientific methods, with the purpose of supplying new knowledge that the voicers will then use at their discretion and according to their personal taste.
One of the steps that has been analyzed concerns the investigation of the differences between the practices of open-toe and closed-toe voicing. Open-toe voicing is a technique by which flue pipes are voiced with their toe hole completely open, thus achieving continuity between the size of the toeboard hole and that of the pipe foot. With this technique, the pipe toe opening is not used to control the volume of sound that the pipe produces. On the other hand, with the technique called “closed-toe voicing” the volume control in the pipe sound is achieved by means of adjusting the diameter of the pipe toe opening.
It is the opinion of many that the difference between the two techniques merely represents a choice in the method for controlling the sound volume of pipes and that there are few and marginal effects on the quality of sound. If the volume can be well equalized by closing the pipe toes, why choose to avoid such practice? Even the first, partial results of the investigation are proving that such an assumption is an oversimplification. The two methods produce different tonal results, which can be detected and measured.
An experimental session was called in April 2009 at the Fraunhofer Institute in Stuttgart. The participants spent two solid days investigating a number of metal pipes specially built for the experiment. The research took place in a very sophisticated structure: a huge anechoic room of almost 2,000 cubic meters in volume. The test “floor,” a steel grille placed at mid-height (20 feet from floor level), housed the several people involved in the experiments, plus all of the needed equipment: sophisticated pressure sensors (along with a less sophisticated old-fashioned wind gauge), computers, sound pressure detectors, state-of-the-art microphones, etc.
The group of researchers included Dr. Judit Angster, head of the Research Group of Musical Acoustics and Photoacoustics of the Fraunhofer IBP; Prof. Andras Miklos, director of the Steinbeis Transfer Center of Applied Acoustics and a world-famous researcher in the field; Johannes Kirschmann, voicer and restorer of the firm Mühleisen of Leonberg, Germany; Francesco Ruffatti, tonal director and head voicer of Fratelli Ruffatti of Padova, Italy; and Thomas Trommer and Maria Cabanes Sempere, scientists at the Fraunhofer IBP.
During this intensive session, two sets of pipes, one of Principal scale and one of Open Flute scale, were analyzed. Each set was made of four identical pipes, two of them voiced with the open-toe and two with a controlled-toe opening. To reduce the risk of subjectivity, each voicer worked on and prepared one open- and one closed-toe pipe. The same procedure was repeated at three different wind pressures, ranging from 70 mm water column, just slightly less than 3 inches, to about 170 mm, or slightly less than 7 inches. Pipes were voiced with no nicking at the languids, but further investigations were carried out also with nicked languids in different configurations. All pipes in each set and for each trial were voiced to equal, instrumentally measured sound volume.
The wind pressure was measured not only inside the windchest but also inside the pipe toes of both the open- and closed-toe pipes.2 The sound of each pipe was also recorded simultaneously but separately at both radiating points, i.e., at the mouth and at the top of the resonator. In addition, the “mouth tone”3 was also recorded from each pipe at each step.
A huge quantity of data was collected, which is currently being analyzed. During the test session, however, several interesting phenomena could already be observed. To everyone’s surprise, it was noted that the wind pressure inside the pipe foot in open-toe pipes showed an average pressure drop of 10% or less from the original pressure inside the windchest, while in the closed-toe pipes, even though these were still fairly open, the pressure drop was about 40 to 50%. A further immediate difference was detected in open- versus closed-toe pipes: under equal conditions, the “wind noise,” a natural component of the pipe sound that the voicer normally tends to reduce or eliminate, was by far more noticeable in closed toe pipes. This is not at all an irrelevant difference: in practical terms, it means that pipes voiced with closed or partially opened toes will require a heavier presence of “nicks” at the languids in order to control the wind noise, and this in turn will determine significant modifications to the structure of their sound.
The final results will be presented, with scientific data and measurements, to the project participants in the near future. These are occasions where the various organbuilders share experiences and learn from the scientists, an invaluable help to modern organbuilding.
—Francesco Ruffatti
The scientist’s viewpoint
Organ building is a traditional craft, which entails a valuable body of knowledge passed from generation to generation and which therefore should be preserved. Nevertheless, innovative design methods and technologies can be applied in the daily practice of this craft in order to optimize the design and production of organs, without endangering the valuable traditions inherent to their fabrication. The organbuilding firms that are taking part in the European research projects recognize that the quality and the effectiveness of their work can be considerably enhanced by adopting scientific and technological innovations into their craft.
In the current pipe project, before starting applied research, it was necessary to carry out fundamental research to reach a better understanding of the physics of flue pipes in organs. Furthermore, some special tools had to be developed, including special software for the analysis of pipe attack and stationary sound. The measurements were carried out in the anechoic room of the Fraunhofer IBP, where an acoustic-free field could be achieved. Here the pipe sound can be detected without any acoustical influence from the surrounding space.
The pipes were positioned on a functioning model windchest. All the other parts of the wind system, like reservoir and blower, were set outside of the room so that the sound detection would not be disturbed by any noises (Figure 1). The sound of individual pipes was detected by changing parameters one at the time, in order to evaluate the physical effect of single voicing steps. The evaluation of the experimental results is currently being carried out with the help of the above-mentioned special software.
The selected flue pipes that are the object of the research are being analyzed from the standpoint of the physical features of their steady sound spectrum and of the analyzed onset of the sound. A stationary spectrum of a flue pipe can be seen in Figure 2. This spectrum shows the most important properties of the sound of flue pipes, some of which are listed as follows:
1. A series of harmonic partials. As is well known from the elements of the Fourier theory in mathematics, any periodic signal has a lined spectrum with several harmonic partials and mostly a complicated spectral envelope.
2. A second series of smaller and wider peaks, which are not harmonically related, but slightly stretched in frequency—these peaks are at the frequencies where the sound will be amplified by the pipe body (acoustically called pipe resonator).
3. A frequency-dependent base line—this is the characteristic noise spectrum of the air flowing out of the flue.
An example of attack transient of an organ pipe of the Diapason family can be seen in Figure 3. Three phases can be subjectively distinguished in the attack of flue pipes.1 These parts cannot be entirely separated in time because they overlap quite broadly. Therefore, it is better to refer to them as three components, which start almost simultaneously, but develop at different rates. These three components can be characterized as follows:
• Forerunner. This is the sound heard first. It is very difficult to describe. It may have a pitch, but sometimes no pitch can be assigned to it. Several different terms are used for this component, such as chiff, ping, hiss, cough, etc.
• Appearance of a pitch. The second component in the attack usually has a pitch close to the pitch of a higher harmonic partial. This component is very important for certain stops. For example, for several diapason stops the second or the third harmonic can be heard preceding the fundamental.
• Onset of the fundamental. The third parameter of the attack is the rise time of the fundamental. For stops of the flute family, this rise time is very short, whereas it is very slow for stops of the string family. As the fundamental grows, certain components of the attack simultaneously become weaker.
The presence of the first two components is not compulsory in the attack. Moreover, the voicer can seriously influence the attack by producing, according to his taste, a faster or slower speed, a more or less pronounced forerunner, brighter or more fundamental sound, etc. It is worth mentioning that sometimes one or more partials are quite strong at the beginning of the attack, but become weaker in a later phase of the development of sound. The measurements show that the perception of the attack can be assigned to measurable properties.
The three parts of the attack can be clearly detected in Figure 3. The forerunner appears in every partial, implying its broadband nature (chiff). Then the partials start to grow; the fastest component is the sixth one. After a while, the second will be the strongest; it dominates the attack in the 35–40 milliseconds domain. The fundamental slowly overtakes the second, which becomes slightly weaker as the fundamental rises.
It can be assumed that the presented characteristics of the attack in flue pipes are related to the basic physical properties of the pipes. These relations will be investigated also in the case of voicing with open and closed toe. In Figure 4 another three-dimensional representation of an analyzed onset (attack transient) of a flue pipe is shown. In this case also the time function of the noise between the partials can be observed.
One of the many tasks of the project is the investigation of the advantages and disadvantages of the voicing methods with an open-toe and with a controlled-toe opening. In doing so, an aspect that has been analyzed from a scientific viewpoint deals with the radiated sound power (“volume of sound”) as a physical parameter.
The values of the pressure and flow are indifferent from a physical point of view, since the same sound power can be achieved by
• large foot pressure and small flue area (voicing by open toe)
or by
• small foot pressure and large flue area (voicing by closed toe).
The sound power depends on the air volume, which is proportional to the flue area and to the square root of the wind pressure in the toe. The pressure in the foot is constant in the case of an open toe; consequently there is only one parameter, the flue area, which can be varied by the voicer. By closed-toe voicing, the wind pressure in the foot can be changed, e.g., in this case two parameters can be set: the wind pressure and the flue area.
There is one more difference that must be mentioned. In a closed-toe pipe, a cross-sectional jump in the flow occurs at the foot hole through which flow noises can be generated. As the measurement results show in Figure 5, the noise level in the pipe sound is lower in the case of voicing with an open pipe foot.
The above are only a few and partial examples of the thorough investigation that is being carried out to evaluate the different aspects and characteristics of the open-toe and closed-toe voicing methods. Their influence on the attack transients will also be investigated.
A great advancement in the research process has come from technology that allows one to see the air flow pattern at the pipe mouth. A plexiglass “window” was created in the pipe, and air mixed with smoke was utilized to activate the pipe. By means of sophisticated equipment, involving a laser light source and a high-speed camera, it has been possible to film the movement of the air flow (see illustrations). The process is the work of scientists Hubert Ausserlechner, Fraunhofer Institute for Building Physics (IBP), Stuttgart, and Margit Liehmann, Fraunhofer Institute for Chemical Technology (ICT), Pfinztal.
In addition to the subject above, the research program has already produced excellent results in examining wooden pipes, open and stopped, of different shapes, with the aim of scientifically calculating the best shape from the standpoint of the efficiency of their air column. In addition, specific research will be aimed at finding efficient solutions for the tonal transitions between stopped and open pipes, or between pipes of different shapes and materials within the same rank. This is not an easy task, but a very exciting one, which can bring immediate and tangible results to the day-by-day work of the organbuilders involved in the research.
—Judit Angster
Common reference projects for cooperation for both authors—European CRAFT (Co-operative Research Action For Technology) projects within the framework of Brite-Euram III program:
1. “Development and Modernization of the Wind Supply Systems of Pipe Organs“ (BRST-CT98-5247)
2. “Advanced Computer Designed Open Wind Systems for Pipe Organs” (G1ST-CT2001-50139)
3. “Development of an innovative organ pipe design method” (G1ST-CT-2002-50267)
4. “Innovative Design Method for Matching the Pipe Organ to the Acoustics of the Room” (COOP-CT-2005-017712)
5. “Innovative Methods and Tools for the Sound Design of Organ Pipes” (FP7-SME-2007-1, Research for SMEs – 222104) (current project)