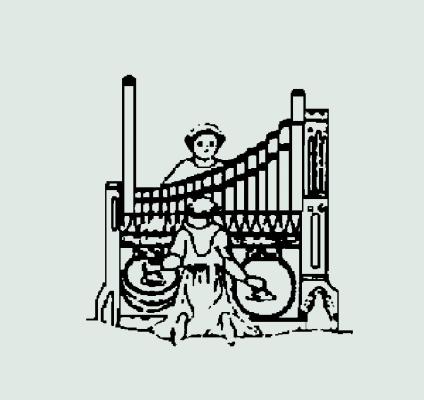
Technology widens the rift.
The other day while running around the house getting ready for work, I heard snips of a story on National Public Radio about the death of Australia’s last veteran of World War I. I missed the man’s name and didn’t hear how old he was, but it’s safe to guess that he was born sometime around 1900. I reflected on the dramatic march of history encompassed by his lifetime, and I recalled a conversation with my grandfather shortly after astronaut Neil Armstrong stepped off a metal ladder onto the surface of the moon. That wise and lovely old man pointed out that his lifetime spanned a comprehensive history of transportation from horse-drawn carts to space travel.
As I write this afternoon, I type my thoughts into a laptop with a twenty-two gigabyte hard drive. I’m no computer historian, but I’m sure that NASA didn’t use a machine as powerful as mine to guide Mr. Armstrong’s route. In fact, I suspect that a lot of the calculating was done with slide rules. My work with the Organ Clearing House involves the management of thousands of photographs so my twenty-gig hard drive is full. I solved that problem by purchasing a sixty-gig supplemental drive. It’s the size of a Band-Aid® box and cost about $150. Navigation involves spherical trigonometry. It’s tricky enough to do those calculations on earth, crossing an ocean for example—it’s exponentially more complicated to navigate between celestial bodies when one is orbiting the other and both are orbiting the sun. How can it be that I need more computing power and memory to manage my organbuilding career than was available for celestial navigation forty years ago?
When President Richard Nixon was defending himself against an impeachment inquiry in the early 1970s, he and those around him were manipulating transcripts of the infamous taped conversations that were being used to implicate him. We read that it took a platoon of secretaries working through the night to retype a transcript in time for a court-appointed submission deadline when a passage was to be deleted in the interest of deceiving the public. This afternoon when I look back on a previous paragraph and have second thoughts all I have to do is highlight and delete. How can it be that I have more stenographic power on my desk than the collective resources of the Nixon White House?
Where are we, anyway?
When I was growing up I loved riding in the car watching the countryside go by. After a childhood and adolescence of looking out the window while your parents did the driving, you’d have a good idea of where you were going when you finally could drive yourself. But now when you shop for a new car you’re surprised to see how many models are supplied with video screens and DVD players. Of course we need those video systems to keep the kids quiet so we can talk on the phone. The logical continuation of this illogical progression is that we can anticipate a generation of new drivers who have no idea where they are going. They’ll have to be taught the meaning of a stop sign or a traffic light. They might not know the way from their home to their school. And they’ve been deprived of thousands of hours of conversation with their parents, siblings, and friends. The good news is that car makers have anticipated this problem. Long before those lost young drivers sit behind the wheel for the first time they’ll be used to satellite navigation. Why strain your eyes looking out the window when you can have a pixilated map on a dashboard screen?
Several years ago my son participated as a crew member competing in a popular annual sailboat race from Cape Cod to Bermuda. There were around a hundred-eighty boats from many different classes so the race officials used a handicap system to level the field, allowing slower boats a mathematical advantage. A further feature of the handicapping system allowed an advantage to those skippers who navigated by the stars without the aid of global positioning satellites and other sophisticated devices. Imagine using a sextant to figure out where you are in mid-ocean when for a few hundred dollars you can have an electronic gizmo that would do it for you. If you’re going to go to all that trouble to know how to do something, shouldn’t you be rewarded for it? (By the way, Mike was in a boat with a sextant!)
In an Op-Ed column in the New York Times of Sunday, October 16, 2005, Pulitzer-prize winning biographer Edmund Morris commented on the recent discovery of the original manuscript of Beethoven’s transcription for piano, four hands of his Grosse Fuge, originally written for string quartet. Mr. Morris began the column by saying that his first reaction to hearing this news was
an aching desire to see it. . . . Beethoven’s manuscripts are revelatory, because he was an intensely physical person who fought his music onto the page, splattering ink, breaking nibs, even ripping the paper in the process. Not for him the serene penmanship of J. S. Bach, whose undulant figurations sway like ship masts over calm seas, or the hasty perfection of Mozart, or the quasi-mathematical constructs of Webern. Their writing is the product of minds already made up.
As he continues, Mr. Morris laments society’s progress away from the authentic process of creating art:
It is already a given that many young architects can’t draw, relying on circuitry to do their imaging for them. . . . Recently my wife and I bought a country house designed by just such an architect. It looked great until we discovered that the main floor sagged in the middle because it lacked the kind of central support that a child, 40 years ago, would have sensed was necessary in the foundation.
Forty years ago, I could have been that child. I credit much of my understanding of load-bearing support, hoisting and rigging, and mechanical advantage to Christmas packages that contained Erector Sets®, Tinker Toys®, Lincoln Logs®, or Legos® just the way I base my knowledge of local geography and my sense of direction on looking out the window of the car when I was child. (Now my wife accuses me of navigating by steeples because I can find my way through unfamiliar neighborhoods using as landmarks the distant steeples of churches where I have worked.) Have you heard about the structural principal of the triangle as a rigid physical form? Assemble a square using four Erector Set® beams of equal length, four bolts, and four nuts. You’ve made a form that you can easily collapse into something like a straight line. Add another piece to form a diagonal across the square. Now it’s two triangles and you can’t collapse it. Simple. (The same grandfather showed me that when I was little.) You don’t need an engineering degree or a computer with a CAD (computer-aided-design) program. You learned it the simple way and you’ll never forget it. Drive past a construction site where there’s a tall crane at work and wonder how it holds itself up. Easy—it’s nothing but a long string of triangles. Arrange your triangles into a succession of three-dimensional forms, and voila: a geodesic dome with thanks to Buckminster Fuller—a simple-to-build roof that can support a load of snow.
They don’t build ’em like they used to.
I know, I know—I sound like an old timer (I really did walk two miles to school every day!). But we who have built our lives around the pipe organ have a unique opportunity to rub shoulders with the good old days. I’ll always appreciate the lessons learned working on a venerable antique organ. While restoring an organ built in 1868 by E. & G. G. Hook, I was particularly impressed by the clarity of the workers’ pencil marks. Those pencils were so sharp that there was no discernable width to the line they left. Mark a mortise on a piece of wood with a pencil you’ve sharpened to a one-molecule point and you’ll certainly cut it just as clean with a chisel. In a modern organ shop, the same oilstone used to sharpen the knife that’s used to sharpen the pencil that’s used to mark the mortise is also used to sharpen the chisel that’s used to cut that mortise. That’s the way it used to be and that’s the way it is!
In fact, they do build them like they used to. Organbuilders commonly celebrate the completion of an organ in the workshop with a party—an “open house.” If you don’t happen to live near an organbuilder, plan ahead when you’re thinking of planning a vacation. Call several organ shops to ask if they have an open house coming up and plan your trip around it. If you’d like some hints, give me a call. You’ll be rewarded not only by seeing a brand-new instrument and meeting other organists and organbuilders, but you’ll certainly be able to get a sense of how ancient honored methods and traditions have been brought into the twenty-first century.
Remember the fundamentals.
When you attend that open house, you might learn the importance of reading the grain of a piece of wood before making it into part of the organ. Look at the end of a piece of wood and you’ll see the pattern we call end-grain. Sometimes you can see the circle of the tree’s rings, sometimes you see a flat pattern where the lines of the grain are parallel with the wide surface of the board, sometimes those lines are perpendicular to the wide side of the board. Draw a cross-section picture representing a tree’s growth rings with concentric circles, draw lines across it that represent boards cut out of the tree, and you’ll be able to figure how a raw tree can be milled to achieve a certain pattern. Why does this matter? Wood warps with the growth rings. In other words, when the end-grain pattern is parallel with the wide side of the board, the board will warp toward the wide side. Consider the keyboards and pallets of an organ with slider chests. If you as the organist could choose, you’d surely prefer to have keyboards warp up-and-down so the individual keys wouldn’t warp into each other and bind, and you’d surely prefer to have the pallets warp side-to-side leaving the gasketed surface flat against the windchest grid avoiding ciphers.
You can choose. Your organbuilder should make the keyboards using wood with the flat grain (often called slab grain). Pallets should be made using wood whose end-grain is perpendicular to the surface of the windchests. Your keyboards won’t stick, and your pallets won’t cipher. Remember the fundamentals.
Those thoughts on end-grain offer a glimpse into the art of making a pipe organ. Organbuilders combine natural and synthesized materials, adapt ancient forms and ideas and combine them with new. They work out the structural requirements of the instrument, computing how its weight is distributed and supported. They fill in the right number of squares with diagonals to make the triangles that keep the instrument’s structure from wobbling or falling. Perhaps their drawings look like Beethoven’s scores, rife with erasures, crossing-out, conflicting ideas. Or do they achieve “undulant figurations” (à la Bach), “quasi-mathematical constructs” (à la Webern) or, God forbid, “hasty perfection” (à la Mozart, whose mind was already made up)?
There’s a big gap between taking the long route and avoiding short cuts—and the right way is somewhere in between. Creating works of art—novels, plays, paintings, statues, musical performances, musical instruments—is strengthened by remembering the fundamentals. All are made possible by the pedagogy, the drudgery, and the excitement of early learning. There’s no substitute for learning the fundamentals. You cannot develop a credible view of the world and your place in it while watching DVDs in the back of the car.