Scott R. Riedel is president of Scott R. Riedel & Associates, Ltd., an acoustical and organ consulting firm based in Milwaukee, Wisconsin.
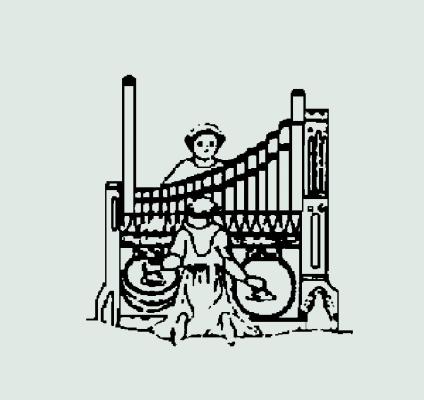
Acoustics in the Worship Space I, II, III, IV, V, VI, VII, VIII, IX, and X have appeared in The Diapason in the May 1983, May 1984, January 1986, May 1987, April 1988, April 1990, July 1991, May 1992, April 2009, and December 2012 issues, respectively.
This article will explore some of the architectural features that are critical to the good musical and technical design and care of an organ. Factors important to achieving an artistic, reliable, and long-lasting organ installation include a supportive acoustic environment, appropriate technical infrastructures, and reasonable protection against damage.
First, an “organ” must be defined. For the purposes of this article, an organ is a musical instrument that produces tone from wind-blown pipes. The “organ” comprises the pipes, wind chests, wind-regulators, expression boxes, and devices that operate in consort to produce musical sound. The “console”—the cabinet that contains keys, pedals, stop controls, and other operating devices—is the “control panel” of the organ, but the console alone is not “the organ.” It should be noted that some of the principles discussed here will apply to digital instruments (which project tone through loudspeakers) as well.
Important building architectural, acoustic, infrastructure, and placement design considerations for the installation of an organ should include the following:
• An organ is best placed high in a room, at the end of the long axis of the space.
(See photo 1.)
• An organ should be placed to have direct tonal projection to all seating and listening locations. The presence of blind corners, deep alcoves, long balcony overhangs, solid balcony railings, or other features that can obstruct “line of sight” tonal access between organ and other musicians and listeners should be minimized or eliminated. (See photo 2.)
• The organ should be placed behind and higher than choir singers or instrumentalists that it will accompany.
• The organ’s area should offer adequate space for all pipes and equipment to be placed in a configuration that allows unimpeded maintenance access. (See photo 3.)
• Options for the acoustic layout and visual design of an organ include encasement (as shown in photo 4), exposed array (photo 5), or chambered pipes (photo 6).
• Façade pipes are preferred over grilled or latticed chamber tone openings for best tonal egress and projection; any chamber tone openings must be as large and open as possible, with minimal obstruction of tonal egress.
• There should be a direct “line of sight” sound pathway between the console and organ pipes, with no obstruction between the organ and organist/console or any other singers or instrumentalists. (See photo 7.)
• Architectural materials should be primarily hard, dense, and sound reflective and diffusing in order to reinforce, reflect, blend, and project organ tone throughout the listening space. Avoid the use of sound absorbing materials near to an organ.
• The blower and motor must be isolated to eliminate any operating noise from being heard in the listening space. (See photo 8.)
• If the blower and motor are located remotely from the organ, airtight ducts are needed to bring wind from blower to organ.
• Interior climate conditions should include stable, even, well-circulated air/temperatures and mid-range humidity conditions.
• Code-compliant electrical power supply and operating system conduits are needed, according to the unique layout needs of any specific instrument.
• Adequate structural support is needed to bear the weight of organ components.
In addition to these acoustic and architectural accommodations for an organ, all other links between the building and organ must be carefully handled. The success or failure of an organ’s installation, maintenance, tone, and durable lifespan can be significantly affected by a building’s other features and systems. Important organ and building interrelationships include the following factors:
• The organ’s space should be dedicated for organ equipment exclusively. Other apparatus should not be placed onto or near to organ components. The organ’s space should not be the route or passageway to other areas of a building such as access to roofs, attics, or other mechanical equipment rooms. Significant damage can be caused to an organ by equipment or uninformed personnel. Situations to beware of include:
– HVAC ducts run through organ chambers or laid on maintenance walkways can make tuning access difficult or impossible (as shown in photo 9).
– Fire sprinkler pipes that are drilled and installed through the center of a windchest will ruin
the windchest.
– Sound, video, or alarm system and communication cables routed through organ spaces or attached to organ windchest bottom boards and legs will make instrument maintenance access difficult or impossible. (See photo 10.)
– Organ pipes can be crushed and ruined by the presence of ducts or cables and those who install them (as shown in photo 11).
– Leaving an organ case or chamber unsealed and unprotected during a building renovation project exposes the instrument to harmful dirt and dust that is expensive and difficult to clean.
– Storing heavy articles on a blower or static regulator can result in damage and adversely affect wind pressure. (See photo 12.)
– Obstructing the blower air intake portals or vents will cause loss of wind pressure and possible permanent damage to the blower, or even fire.
• Drain pipes, water pipes, HVAC ducts or vents, and any other similar building system components should not be routed through organ spaces. These items can obstruct organ equipment, maintenance access, and tone projection. There can also be potential damage to the organ from heat, cold, dirt, leakage, and condensation, etc., from such systems.
• HVAC air exchangers, cold air returns, or supply grilles should not be placed within organ spaces or chambers. Organs require consistent and clean air and climate conditions. The placement of HVAC components within organ spaces can create excessive amounts of dust and dirt, as well as turbulent air and unstable temperatures. Low humidity levels will hasten leather decay and wood damage. Organs benefit from evenly circulated air of stable or slowly changing climate conditions. (See photo 13.)
• Organ infrastructure such as wind ducts, conduits, and electrical power supply lines should be dedicated exclusively for organ use. Running sound, video, alarm or other systems’ cables or power lines within organ conduits can cause operating difficulties and failures.
An organ is a musical instrument—truly a piece of art—and is also a complex set of unique mechanical and electrical devices. The instrument can also be costly to create or repair if damaged. Other than the church building, it may be the single most expensive item a parish owns. An instrument should be treated with respect and given proper care. To perform well, it should not be unduly limited in its location, layout, or access. Most importantly, an organ’s space and components should not be imposed upon by other building systems, features, or activities. An organ will provide good, durable, and economic service for decades (as can be seen in photo 14), given appropriate care and if left undisturbed.