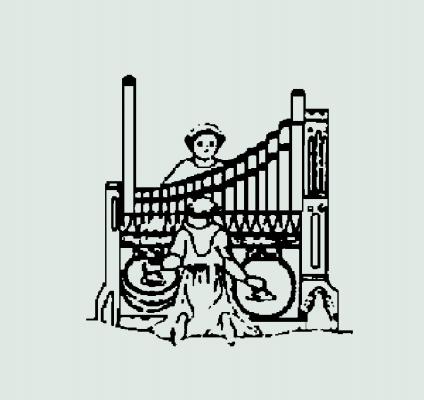
Preface
The 1863 E. & G. G. Hook organ, Opus 322, is not only one of the best preserved of the earlier instruments of that firm, it had the good fortune to be placed in the superlative acoustics of the Church of the Immaculate Conception in Boston, Massachusetts. Rooms with long acoustical reverberation are rare in the United States. Rarer still is a room where all frequencies of sound die away cleanly at a similar rate. At Immaculate Conception the clean diffusion of sound without slap echoes was enabled by a profusion of complex cornices and a coffered ceiling with intricate ornamentation; it is a wonderful example of a fusion of form and musical function.
Designed by noted architect Patrick Charles Keely, the classical façade of the church is executed in granite. The organ resided directly behind the windows above the main doors. The Church of the Immaculate Conception is situated in a historic district of Boston. Nearby elegant row houses reflect an age when architectural design valued a balance of form and the texture that cornices, corbels, and moldings bring to a structure. These classical elements live and reverberate into the new millennium.
Detailed documentation of such a universally acclaimed organ is important for several reasons. We can learn how the Hooks designed their organ to suit the acoustics. We can make useful comparisons with other organs and learn how this Hook differs from other styles of organ design. And perhaps most importantly, we can document this organ for posterity. Organs are consumed in wars and fires; they are replaced or modified with the changing tastes of time; and they never survive a restoration without changes. In a quirk of fate that makes this documention all the more valuable, the organ was dismantled in 2008 and placed in storage for Boston College. Plans by developers now exist to convert the Church of the Immaculate Conception into condominiums.
In June of 2000 the Jesuit Urban Center and its director, Fr. Thomas Carroll, SJ, invited the author to reside with them for a week at the Church of the Immaculate Conception with the goal of acquiring detailed data on the Hook organ. The author immersed himself in this work to such a degree that he often lost track of the passage of time. The resident Jesuits would ascend to the organ loft to remind the author that it was time to end a long day of work, promising good conversation and good libations as a reward, to which the author always happily acquiesced. The following study is an analysis of the data taken while a guest of the author’s most generous hosts.
The data showed that the Hook organ is in remarkably original condition, primarily the result of its careful maintenance by many generations of the Lahaise family. The data also revealed some crude interventions originating from the repitching of the organ and the 1902 installation of the Solo division, all of which are reversible.
Current stoplist
Great
16′ Open Diapason
8′ Open Diapason Forte
8′ Open Diapason Mezzo
8′ Viola da Gamba
8′ Clarabella
4′ Octave
4′ Flute Harmonique
3′ Twelfth
2′ Fifteenth
III Mixture
V Mixture
VII Cymbal
16′ Trumpet
8′ Trumpet
4′ Clarion
Swell
16′ Bourdon
8′ Open Diapason
8′ Stopped Diapason
8′ Viol di Amour
8′ Voix Celeste
8′ Quintadena
4′ Octave
4′ Violina
4′ Flauto Traverso
2′ Flautino
V Mixture
16′ Contra Fagot
8′ Cornopean
8′ Oboe
8′ Vox Humana
4′ Clarion
Choir
16′ Contra Dolce
8′ Open Diapason
8′ Melodia
8′ Gedeckt
8′ Viola
8′ Dulciana
4′ Octave
4′ Fugara
4′ Hohlpfeife
4′ Flauto Traverso
2′ Piccolo
8′ Clarinet
Pedal
32′ Contra Bourdon
16′ Open Diapason
16′ Violone
16′ Bourdon
12′ Quint Floete
8′ Violoncello
8′ Flute
16′ Trombone
8′ Trumpet
Solo
8′ Open Diapason
8′ Concert Flute
4′ Flute Harmonique
8′ Tuba Mirabilis
8′ Orchestral Oboe
8′ Orchestral Clarinet
4′ Tuba Octave
Casework and façade
Built in 1863, the Hook organ casework is constructed of pine, not a hardwood. Perhaps the Civil War took its toll on the availability of materials. The case façade was designed by the church architect, Patrick C. Keely.1 Although it employs extreme over-lengths in the façade pipes, the case and nave are a successful fusion of the architectural style.
There are seven flats of pipes in the façade. The flats at the extreme sides contain three dummy pipes each. The Hooks utilized bass pipes from both the 16′ Open Diapason and the 8′ Open Diapason Forte in the façade. A few bass pipes that would normally be a continuous part of the façade were placed just behind the façade on offset boards in an effort to keep a normal progression of widths when using the pipes of two different stops. This resulted in some very large overlengths with many cutouts at the back of some pipes. Here is the order of speaking case pipes, facing the case, from left to right:
9 pipes: 8′ F#, 8′ E, 8′ D, 8′ C, 16′ G#, 16′ c, 16′ d, 16′ e, 16′ f#;
3 pipes: 16′ c′, 16′ a#, 8′ A#;
9 pipes: 16′ A#, 16′ F#, 16′ E, 16′ D, 16′ C, 16′ C#, 16′ D#, 16′ F, 16′ A;
3 pipes: 8′ B, 16′ a, 16′ b;
9 pipes: 8′ G, 16′ d#, 16′ c#, 16′ B, 16′ G, 8′ C#, 8′ D#, 8′ F, 16′ f.
The form and presentation of the data
Pipe measurements, which include enough data to reconstruct the voicing, were taken on selected pipes of the principal chorus of the Great division. Measurements taken by the author were entered into a laptop computer with the gracious help of Paul Murray, a volunteer at the church. The computer was set up within the expansive casework to make efficient use of time.
A total of 25 measurements and notes were taken on each of the selected pipes of the principal chorus; 34 measurements and notes were taken on a Clarion reed pipe. While this may seem excessive by normal practice, the standard for this type of documentation was pioneered by Pierre Chéron in his classic work on the Isnard organ at St. Maximin, L’Orgue de Jean-Esprit et Joseph Isnard dans la Basilique de la Madeleine à Saint-Maximin, France.
The scaling sheets devised by Mr. Chéron were adapted by the author to a spreadsheet.2 Analysis of that data enabled a detailed understanding of the changes made to the 1863 organ. The missing gaps in the data reflect the inability to gain easy access without risk of damage to some pipes, along with the limitations of time.
The author has shown how this data can be portrayed to advantage in his book, The Sound of Pipe Organs, published in 2012.3 This book describes models which can be used to intuitively compare the scaling and voicing of different organs, allowing us to visualize and understand the differences. The reader is referred to this book for a deeper understanding of the models which are presented in this study of the Hook.
The basic data set to describe scaling and voicing must include, at a minimum, pipe diameters, widths of mouths, heights of mouths (“cutups”), diameters of foot toe holes, depths of mouth flueways, and treatment of the languids. The data in this study of the Hook principal chorus is graphically presented side-by-side with a graphical reduction of the data compiled by Chéron from the famous Isnard organ at St. Maximin, France. This is an instructive comparison. The two organs are of similar size and were designed for similar acoustics, but they represent very different tonal ideals ranging from the late 18th-century French Classical traditions of the Isnards to the fully Romantic middle 19th-century traditions of the American Hooks.
Normalized data is presented for inside pipe diameters, mouth widths, and mouth heights (cutups). The tables in Figure 1 show how the raw data was converted into normalized data.
Scaling and voicing
Pipe diameters
The Normal Scale of pipe diameters is a way to visualize relative power, where a flat line from bass to treble will produce relatively constant power. Pipes with data extending higher in the graph will produce more power. Each half tone on the vertical scale represents 0.5 dB of power. Interested readers can refer to The Sound of Pipe Organs, pp. 8–32 for a discussion of the underlying theory.
With the exception of the narrower Mixture V and Cymbal VII, the chorus of the Hook organ in Figure 2 is compressed, i.e., the foundation and upperwork stops have a similar, or “constant,” scale, and the trebles are relatively flat in scaling. In stark contrast in Figure 3, the Isnard scales become narrower as the pitch of the stops ascend, while the 4′ and Mixture scales widen dramatically in the treble.
Wind pressure has a very large effect on power, but fortunately the wind pressure of the Hook organ at 76 mm water column is close to that of the Isnard organ at 83 mm. Power balance differences in these two organs result from differences in the pipe construction (pipe diameters and mouth widths) and differences in the voicing parameters (toe hole diameters and flueway depths).
Mouth widths
The Normal Scale of mouth widths operates just like the pipe diameters, where a flat line from bass to treble will produce relatively constant power. Pipes extending higher in the graph will produce more power. Each half tone on the vertical scale again represents 0.5 dB of power.
Mouth widths are often a better indicator of power balances than pipe diameters, simply because mouth widths can be designed to vary within the same diameter of a pipe. Narrower mouths will produce less power, even if the pipe diameters are wide.
The chorus of the Hook organ in Figure 4 is again compressed, much like the pipe diameters in Figure 2. The Mixture III is scaled as wide as the foundation stops. Note how the Cymbal VII and the Mixture V are the only narrow upperwork stops. Furthermore, the mouth scales of those two mixtures actually descend from bass to treble. The Cymbal VII was made and installed in 1870 by William A. Johnson,4 and it is representative of his typical chorus scaling with wide foundations and much narrower upperwork.
In Figure 5 the scales of the 4′ and Mixtures on the Isnard organ ascend dramatically from 1′ pitch to 1/8′ pitch. Isnard’s intent here is two-fold: the upperwork not only ascends in scale for an ascending treble, it is also scaled to compensate for the losses of power due to the atmospheric absorption of sound over long distances at higher frequencies. Interested readers can refer to The Sound of Pipe Organs, pp. 13–14, for a discussion of the foundations of this very important scaling principle.
These two graphs show the basic differences in the tonal balances of these organs, where the Isnard exhibits a well-balanced full spectrum of frequencies, while the Hook is tailored for warm and powerful foundations with a restrained full frequency spectrum.
Notes and Credits
All photographs, tables, graphs, and data are by the author except as noted.
1. Owen, Barbara. “A Landmark within a Landmark: The 1863 Hook Organ,” undated typescript.
2. Excel files with all raw data taken on the Hook and the spreadsheets that produced the graphs and tables may be obtained at no charge by e-mailing the author at: [email protected].
3. McNeil, Michael. The Sound of Pipe Organs (Mead, Colorado: CC&A), 2012, 191 pp., Amazon.com.
4. Huntington, Scot L., Barbara Owen, Stephen L. Pinel, Martin R. Walsh, Johnson Organs 1844–1898 (Richmond, Virginia: OHS Press), pp. 17–18.
To be continued.